The development of hydrogen (H) economy calls for high-performance structural metallic materials that could safely serve in a hydrogen environment. High-strength aluminum (Al) alloys, as the primary choice of metallic materials for structural lightweight design, are notoriously sensitive to environmentally assisted cracking and highly subject to H embrittlement, like most high-strength metals such as steel and titanium alloys. H-tolerant and high-strength Al alloys are urgently needed, while the microstructural design for hydrogen resistance is still in lack. Recent near-atomic-scale analysis revealed that some second phase particles in Al alloys are capable of trapping and storing H atoms, preventing H atoms from grain boundary segregation and suppressing hydrogen embrittlement (HE) effectively. These findings shed light on potential application of second phase particles to resist H-degradation. However, the principles to select and engineer high-H-trapping second phase particles are yet unclear.
Now an article published online in Nature answers to above questions by dispersing a type of complex metallic phase nanoprecipitates in Sc-added Al-Mg alloys, which leads to unprecedent HE-resistance among the high-strength Al alloys. This work was jointly carried out mainly by Profs. Jun Sun and Gang Liu’s group at Xi’an Jiaotong University, China, Prof. Baptiste Gault’s group at Max-Planck-Institut für Eisenforschung, Germany, and Profs. Yuan-tao Xu and Xue-jun Jin’s group at Shanghai Jiao Tong University, China. The joint research team found a general trade-off relationship between the H-binding energy (Eb, representing a thermodynamic driving force to absorb H atoms) and dispersion number density (N, representing a kinetic process to maximize H-partition) of second phase particles that naturally form in the high-strength Al alloys (see Fig. 1). A high Eb and a high N, although being simultaneously required for an excellent H-resistance, are mutually exclusive based on the known second phase particles. It is thus expected that an unusual second phase with a superhigh Eb could be explored, and at the same time, be dispersed within the grain interior as nanoparticles.
The joint research team innovatively proposed complex metallic phases working as potential H-trapping sites in the Al alloys. The complex metallic phases (CMPs) are characterized by the presence of icosahedral coordination, large lattice constants and correspondingly a number of atoms per unit cell typically ranging from tens to thousands. In particular, the Al3Mg2 phase, with a b-Samson structure, is one of the most complex intermetallic structures. Its cubic elementary cell contains 1168 atoms that are distributed over 1832 atomic positions, with partially occupied sites generating split positions that induce structural disorder (Fig. 2). Since almost one third of atomic sites is only partially occupied (structural vacancies) or are split positions (structural half-vacancies), the Samson-Al3Mg2 phase can be expected to have a high solubility for H and act as an efficient trap. Actually, DFT simulations reveal a record Eb of greater than 0.9 eV/atom inherent to the Samson-Al3Mg2 phase. Nevertheless, due to its high nucleation energy barrier, the Samson-Al3Mg2 phase nucleates readily at grain boundaries and coarsens to a large size, especially in high-Mg Al-Mg alloys. It is challenging to engineer the Samson-Al3Mg2 phase refined down to nanoscale and dispersed within the grain interior.
To overcome the aforementioned bottleneck, the joint research team developed a two-step heat treatment in minor Sc-added Al-Mg alloys, where a high-density dispersion of Al3Sc nanoprecipitates was created in advance during the first-step heat treatment and Samson-structured Al3(Mg,Sc)2 nanophase was subsequently in-situ formed on the Al3Sc nanoprecipitates during the second-step one (Fig. 3). The in-situ solid state phase transformation enables the Al3(Mg,Sc)2 nanophase have a high number density, and the partial replacement of Mg by Sc atoms leads to a further increase in Eb. Interestingly, the core-shell Al3(Mg,Sc)2/Al3Sc nanophase forms only when the Al3Sc nanoparticles are above 10 nm in size, showing a strong size effect. The Al3Sc nanoparticles smaller than 10 nm are free of Al3(Mg,Sc)2 nanophase and make main contribution to strength. The size-dependence is associated with Al3Sc nanoprecipitate incoherency, which leads to local segregation of magnesium and triggers the formation of Al3(Mg, Sc)2. The tailored distribution of dual nanoprecipitates in our Al-Mg-Sc alloy provides a ~ 40% increase in strength and nearly 5-times improved HE resistance compared to the Sc-free alloy and other commercial Al-Mg-based alloys (Fig. 4), reaching a record tensile uniform elongation in Al alloys charged with H up to 7 ppmw. This microstructural design strategy showcases a possible route to increase hydrogen resistance in high-strength Al alloys and could be readily adapted to large-scale industrial production.
More information can be referred to: S. Jiang, Y. Xu, R. Wang, X. Chen, C. Guan, Y. Peng, F. Liu, M. Wang et al: Structurally complex phase engineering enables hydrogen-tolerant Al alloys. In: Nature (2025). DOI:10.1038/S41586-025-08879-2
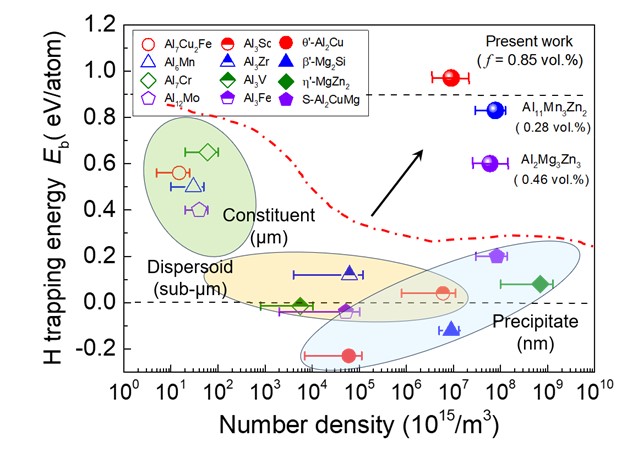
Figure 1 Intermetallic compound particles (ICPs) in Al alloys: a trade-off relationship between H-trapping energy (Eb) and number density, two requisites for mitigating HE resistance effectively. The dilemma is broken in present work through a two-step phase transformation that leads to a high-density dispersion of Al3(Mg,Sc)2 nanophase.
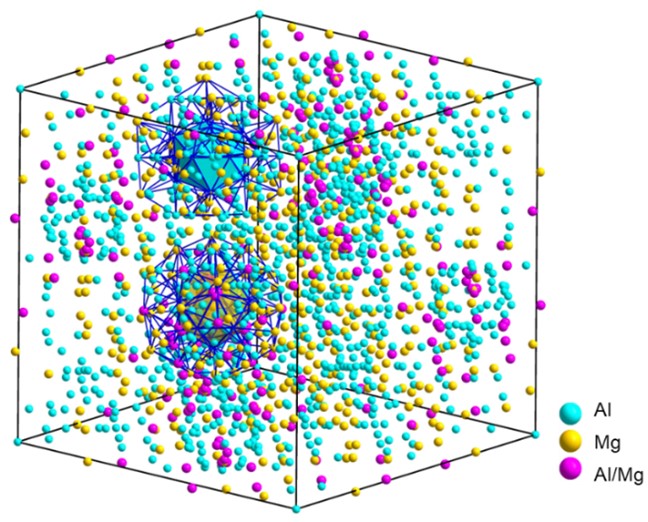
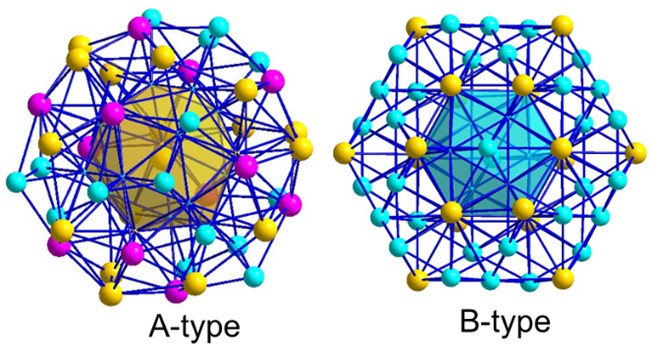
Figure 2 Crystal structure of the cubic Samson-b-Al3Mg2 phase (top), which can be topologically described as an assembly of two types of two-shell nanoclusters, i.e., A-type and B-type (bottom).
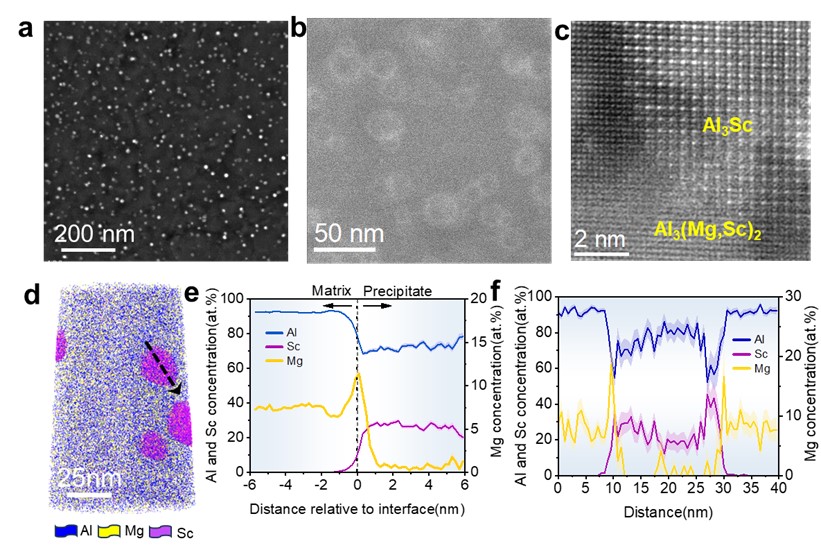
Figure 3 High-density Al3(Mg, Sc)2 nanophase via in situ phase transformation. Representative low-magnification (a) and high-magnification (b) TEM images to show the high-density formation of Al3(Mg, Sc)2/Al3Sc nanophase in the Sc-added Al-Mg alloys. HRTEM image to show the Al3(Mg, Sc)2 nanophase in-situ formed on the Al3Sc nanoprecipitate (c). Representative APT image (d), and composition proximity histogram cross the interface (e) and 1D profile element concentration distributions (f) cross the nanoprecipitate indicated by arrow in (d) manifest a high Mg concentration at the outer “different”phase.
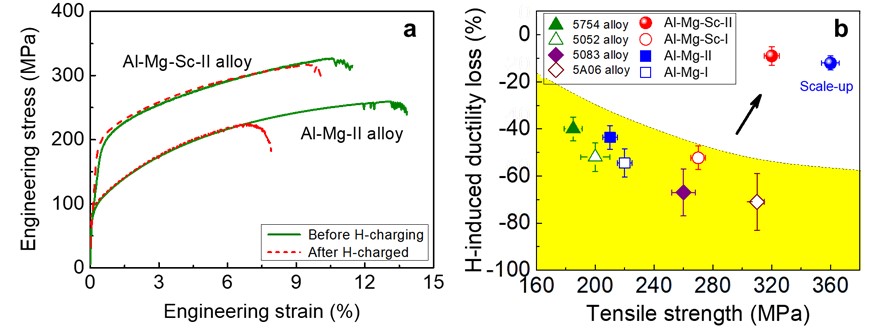
Figure 4 (a) Representative tensile stress-strain curves of the Sc-added and Sc-free Al-Mg alloys before H-charging (solid lines) and after H-charged (dash lines) for comparison (a). (b) H-induced ductility loss: present Sc-added Al-Mg alloy (solid red circle dot) compared with the other control alloys and four commercial 5xxx Al alloys at similarly ~ 7ppmw H-charged.
Paper Link:https://www.nature.com/articles/s41586-025-08879-2