Magnetic materials play a crucial role in spintronics and its applications. Beyond traditional ferromagnetic systems, antiferromagnets have attracted growing attention in the fields of condensed matter and materials physics in recent years, owing to their unique advantages such as ultrafast spin dynamics, precise control schemes, absence of stray magnetic fields, and robustness against external magnetic interference. However, due to the lack of a net magnetization, one of the key scientific challenges in antiferromagnetic spintronics is how to effectively detect and manipulate their order parameter—the Néel vector.
To complement the respective advantages of antiferromagnets and ferromagnets, researchers have recently proposed an unconventional class of antiferromagnets, known as altermagnets. Unlike conventional antiferromagnets, altermagnets exhibit non-relativistic spin splitting, which could lead to finite anomalous Hall conductance. However, such a transport behavior is not a sufficient condition to characterize the altermagnetic order. As a result, developing new methods to detect and distinguish these systems—particularly from a spintronic perspective—remains an open question in condensed matter physics.
Professor Jian Zhou from the Center for Alloy Innovation and Design (CAID) at Xi’an Jiaotong University, along with his collaborators, highlighted that the key to solving this issue lies in clarifying the theoretical relationship between the Néel vector and magnetic symmetry in both conventional antiferromagnets and altermagnets. Building upon their previous theoretical results on nonlinear optical transport and spin torque in magnetic materials (Phys. Rev. Res. 2023, 5, 013001; ACS Nano 2024, 18, 24317), as well as a detailed symmetry analysis of both systems, they proposed a hidden mechanism—photoinduced local spin torque—that can effectively differentiate between these two closely related magnetic orders.
Through a systematic classification of all 122 magnetic point groups, they identified the magnetic groups associated with both conventional antiferromagnets and altermagnets and derived the corresponding magnetic subgroups governing their Néel vector symmetry. They demonstrated that under linearly polarized light, conventional antiferromagnets exhibit pure Néel torque, while altermagnets display both Néel torque and spin canting. Unlike transport-based approaches, this hidden photoinduced spin torque serves as a necessary and sufficient condition, making it a robust method to distinguish among the conventional antiferromagnets, altermagnets, and non-magnetic systems (Figure 1). Guided by this symmetry-based theoretical framework, the team employed low-energy Hamiltonian modeling and first-principles calculations to reveal the role of relativistic effects in the process. They found that light with moderate intensity can induce a local magnetic moment of approximately 0.01 μB, within the detectable range of current experimental techniques, thereby laying a solid theoretical and computational foundation for future experimental validation.
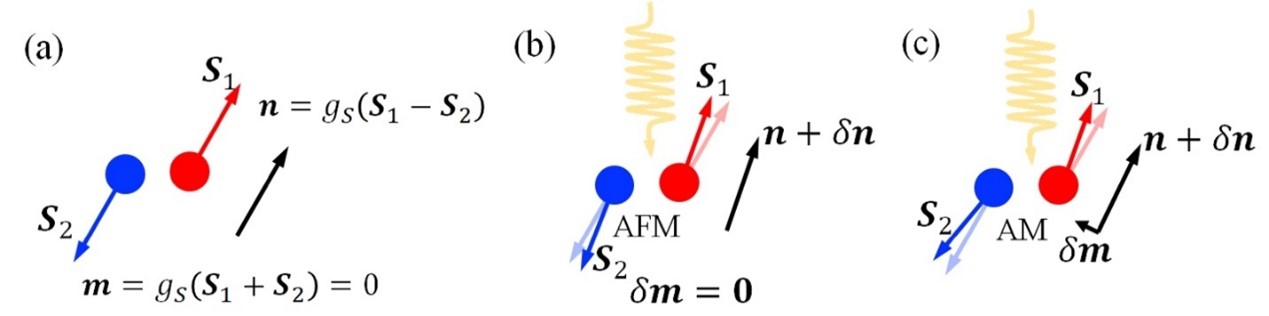
Figure 1. Schematic illustration of the hidden photoinduced spin torque modes for (a) intact, (b) conventional antiferromagnet, and (c) altermagnet under illumination.
This research was recently published in the prestigious journal Physical Review Letters under the title “Contrasting Light-Induced Spin Torque in Antiferromagnetic and Altermagnetic Systems” (Phys. Rev. Lett. 2025, 134, 176902). The study was led by the Center for Alloy Innovation and Design (CAID) at the State-Key Laboratory for Mechanical Behavior of Materials (SKL-MBM), Xi’an Jiaotong University. Professor Jian Zhou and Professor Chunmei Zhang from the School of Physics at Northwest University served as co-corresponding authors. The work was supported by the National Natural Science Foundation of China.
Link to paper: https://journals.aps.org/prl/abstract/10.1103/PhysRevLett.134.176902